Near-Certain Discovery of Quark Matter in Neutron Stars
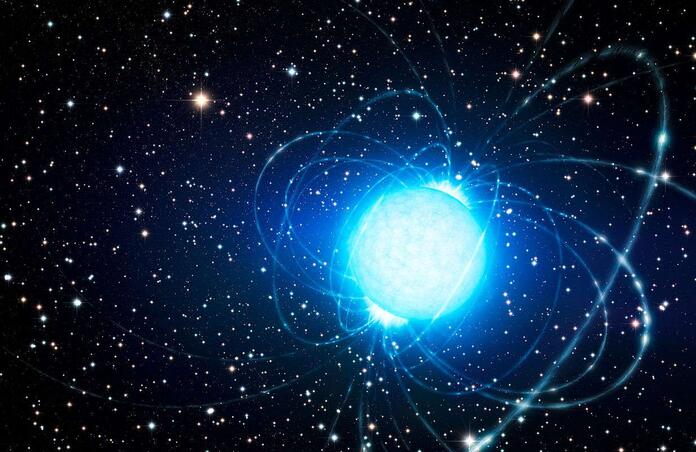
Neutron stars are the most extreme non-black hole environments in the universe, formed from stars over 8 M☉, through a Type II supernova. If the remnants are below the Tolmann-Oppenheimer-Volkoff limit (~2-3 M☉), a neutron star may emerge. These stars achieve hydrostatic equilibrium through neutron degeneracy pressure and forces like the strong nuclear force, resulting from neutronisation mainly by inverse beta decay.
Normally, quarks group in threes as baryons, balanced by the strong nuclear force, which itself is mediated by gluons. However, in extreme conditions this equilibrium can be disrupted. Quantum chromodynamics suggests that at extremely short distances, quarks may interact beyond their typical groups, potentially leading to a phase transition into a so-called quark-gluon plasma. This possibility, whether the quarks form new structures within the cores of neutron stars, remains a key area of research.
In a paper by Eemeli Annala et al, they further explore this possibility, combining astrophysical observations with rigorous theoretical calculations. Through this Bayesian inference, they provide the most robust quantitative measures for the likelihood of quark matter cores, given their observational data, concluding to ~88% certainty that this is indeed the case.
To start, the study leverages ab initio investigations of baryonic-nuclear matter (BNM) and quark matter (QM), which had concluded notable distinctions between their respective equations of state (EoS). While BNM is characterised by the mass scale of the nucleon, QM has been shown to exhibit near-conformal symmetry, meaning the physical behaviour of QM is invariant to changes in the mass of particles. This has notable implications for the values of certain thermodynamic parameters used in this investigation. These allowed for the derivation of a prior probability distribution of the relevant EoS.
Subsequently, these predictions were refined by incorporating data from 12 neutron star targets with reliable mass-radius measurements. These datasets were obtained from various observational facilities, including Virgo and LIGO, as well as data from NICER. From this diverse dataset, a likelihood function, another component in Bayes' theorem, was derived. This likelihood function quantifies the agreement between the theoretical prior and the observed data, essentially assessing the probability of observing the data given the assumptions made in the equation of state.
The study discusses the origin of the remaining uncertainty. It is currently believed that the phase change between NBM and QM constitutes a first-order transition, meaning that there is a discontinuous change in the parameters characterising the thermodynamic state. The most impactful source of error arises as a possibility that if the stellar material undergoes such a phase transition, it may be sufficient to destabilise the system, leading to the gravitational collapse of such stars into a black hole, even for very small QM cores.
Annala et al.'s study significantly advances our understanding of neutron star interiors, proposing with a high degree of certainty the presence of deconfined quark matter in the cores of massive neutron stars. This analysis draws upon conformal symmetry as a key indicator of this phase transition. While the findings are promising, the authors emphasize the need for more lines of evidence to finally and conclusively establish the existence of quark matter in neutron stars.
--
Journal Source: E. Annala et al, Strongly interacting matter exhibits deconfined behavior in massive neutron stars, Nature Communications, 2023, DOI: 10.1038/s41467-023-44051-y
Cover Image: ESO/L. Calcada. CC BY 4.0