Theory Revises the Mechanism of Planetary Formation
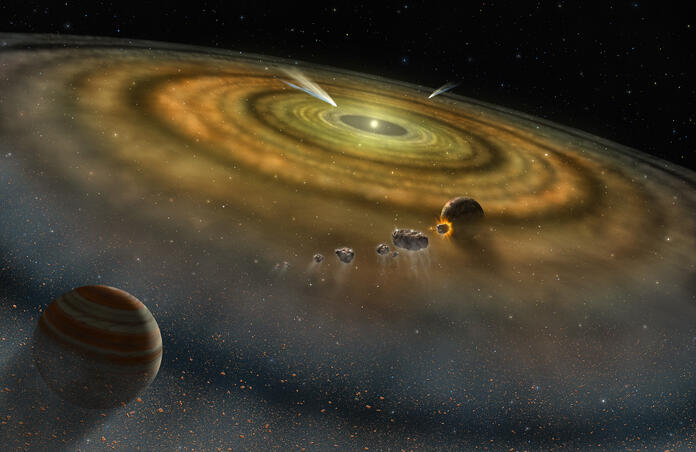
So-called ‘Super Earths’ are described as planets with masses that exceed that of the Earth’s, but remain lighter than the ice giants in our system. These types of planets make up the most prominent proportion of the Milky Ways’s planetary population. However, there remain a few key gaps in understanding with respect to their formation mechanism. These types of planets tend to form very close to their host star. Additionally, while between systems, there is great diversity in the sizes of rocky planets, within systems with multiple, their masses will be very similar. Our current modelling of these systems does not yield the results that can be seen, and so some alternative approach is needed.
Published on the 12th of January 2023, Konstantin Batygin and Alessandro Morbidelli propose an adaptation of the most accepted theory to clarify some more specific aspects of rocky planetary formation. The theory in question describes material within the protoplanetary disk undergoing Brownian motion. These particles will randomly begin coagulating during collisions until they are about one kilometre in diameter. At this point, it is classified as a planetesimal. It is now massive enough to attract further material via mutual gravitational forces.
This revised theory suggests that the formation of such planetary bodies occurs largely according to a similar mechanism through which the four of Jupiter’s moons, Io, Europa, Ganymede and Callisto, were formed. Naturally, however, on a much larger scale. The paper suggests that all planetary formation occurs within a very discrete ring of material around the star, as opposed to throughout the disk. This ring is known as a ‘Planetary Factory.’
Fundamentally, the size and nature of a star’s radiation drives the conditions of its protoplanetary disk, most notably the temperature and viscosity of the gas. The disk forms as material falls near the star at ~0.1 au from the star. The radiation pressure from the star pushes this outward, away from it.
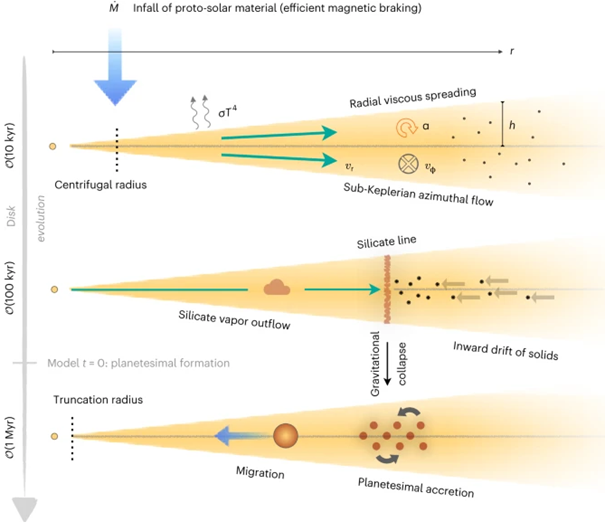
The region of planetary formation is therefore around the radius where silicates undergo deposition from its gaseous phase to solid. Within this radius, silicates are gaseous, but are driven toward larger radii alongside, dragged along by the viscosity of the outflowing gas in the rest of the protoplanetary disk. Beyond this, silicates depose onto dust grains, which begin to grow in mass, and are thus driven towards the centre of rotation. The outcome of this is that silicate material will begin to accumulate at its gas-solid phase boundary, where hydrodynamic occurs between different phases of the material. Here, planetesimals are able to form, as described above.
The planetesimals individually grow to ~100km in diameter, where they now begin to combine into a larger planet. As this occurs, angular momentum conservation causes the larger body to spiral back toward the star. Once they leave the region of planetesimal formation, they will cease to continue growing, which places a limit on their size. The planetary factory is now empty of large bodies and is able to support the formation of further planets. This process will only yield planets of consistent sizes and describes why they tend to be so similar within a system.
The duo have performed a series of simulations, replicating the above parameters. The outcome of these yielded models significantly more similar to what we have been observing in nature. By varying the conditions of the host star, they are able to control the density of the formation ring, with denser rings yielding larger mass planets, but more importantly, a sparser ring yielding a system similar to the inner planets of our solar system.
--
Journal Source: K. Batygin, A. Morbidelli, Formation of rocky super-earths from a narrow ring of planetesimals, Nature Astronomy, 2022, https://doi.org/10.1038/s41550-022-01850-5
Cover Image: Credit: NASA/FUSE/Lynette Cook