The Fastest Growing Black Hole in the Early Universe
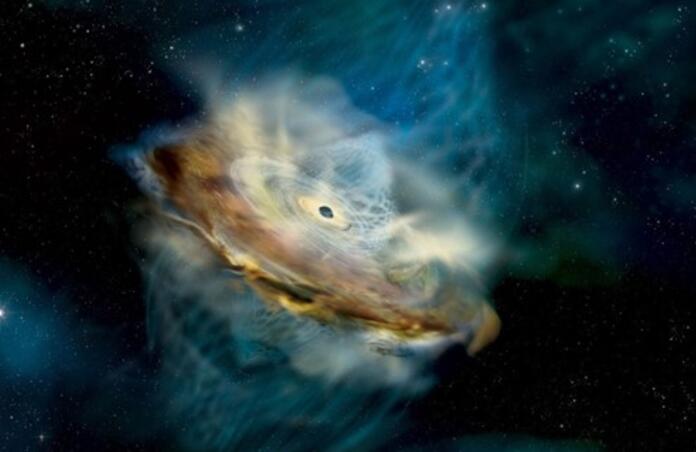
It is not quite understood why we are able to observe supermassive black holes (SMBHs) early in our universe’s history. Leading theories can be separated into two camps. The light-seed theory posits that the earliest black holes are the remnants of the first population of stars, while the heavy-seed theory claims that early black holes arise from the direct collapse of large gas clouds. However, neither can completely explain both the presence and abundance of primordial SMBHs. The key constraint on light-seed models is the Eddington accretion rate, a theoretical limit on the rate at which the mass of bodies can grow, and thus the mass a body can attain within a given period of history.
Now, new observations by the James Webb Space Telescope (JWST) have led to the identification of a small black hole growing much faster than these theoretical calculations. Black holes emit radiation as material within their accretion disks spirals inwards, losing gravitational potential energy. This energy is converted into kinetic and thermal energy, which is then dissipated through interactions between particles as radiation. As such, the rate of accretion is directly proportional to the luminosity of a black hole. Since this radiation will exert an outward pressure on matter, there exists a balanced accretion rate, at which the force exerted by radiation pressure is equal to the central gravitational force. This is the Eddington limit.
A study led by Hyewon Suh identifies LID-568, whose active nucleus contains a low mass black hole at a cosmological redshift of z = 4, presently observed at around 1.5 Gyr after the Big Bang. Based on their measurements, they find that LID-568 is growing around 40 times the Eddington limit for its mass. This is referred to as super-Eddington accretion and requires very specific conditions to achieve.
The study utilises high resolution spectra collected by the JWST and the Chandra X-ray Observatory. By measuring the doppler broadening of key lines in the spectrum, researchers can infer the kinetic energy of the gas, which by the virial theorem is proportional to its gravitational potential. This can in turn be used to determine the luminosity and growth, as previously discussed. This demonstrated that LID-568 had relatively low mass of 7.2 × 106 M⊙ as well as a luminosity 41.5 times greater than its Eddington luminosity.
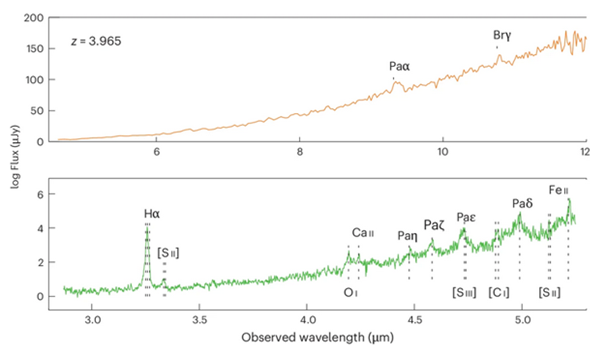
Further investigations into the Hα line of hydrogen provided more detailed descriptions of the gas kinematics. The study used Gaussian fitting techniques to decompose line features into distinct components, isolating a broad central feature and an intense, narrow feature, appearing blue-shifted relative to the cosmological redshift. These features are respectively attributed to the bulk motions of the gas and an outflow from the active nucleus, with speeds reaching as much as 600 km/s.
The study notes a non-spherically symmetric accretion mechanism, instead accreting matter within an anisotropic, disk-like structure, allowing growth to be less-so limited by the spherically symmetric Eddington model. In addition, these observed outflows suppress the growth of stars within the galaxy, which require cool, non-turbulent conditions. This intense outflow injects tremendous amounts of kinetic and thermal energy into the surrounding gas, preventing overdensities from coalescing into clumps capable of initiating fusion. In turn, this allows the gas to be channelled toward the AGN, rather than being used for star formation. Within an already dense early universe, LID-568 is surrounded by enough material to consistently sustain its rapid growth for substantial periods of time.
The study explores the possibility of an intense, super-Eddington phase occurring in most early supermassive systems. This mechanism supports the idea now places light-seed models as a viable pathway for forming primordial SMBHs, as heavy-seed models continue to struggle with the improbability of large gas clouds collapsing directly without fragmentation. However, the study remains uncertain whether this phase occurs only in the densest regions of primordial space, such as galactic protoclusters.
Their findings suggest that LID-568 resides in a region of typical galactic density, with no evidence indicating it belongs to any cluster. This raises the possibility that super-Eddington phases could occur more broadly. However, due to limited data, the study could only detect large protoclusters. Therefore, it’s still possible that LID-568 is part of a smaller protocluster, warranting further investigation into the potential for sustained super-Eddington phases in galaxies throughout the universe.
--
Journal Source: H. Suh et al, A super-Eddington-accreting black hole ~1.5 Gyr after the Big Bang observed with JWST, Nature, (2024), DOI: https://doi.org/10.1038/s41550-024-02402-9
Cover Image Credit: NASA/Aurore Simonnet (Sonoma State Univ.)